Microbially Induced Calcite Precipitation (MICP) is a bio-geochemical process that induces calcium carbonate precipitation within the soil matrix. The process builds up calcite crystals (or aragonite in marine environments) in the spaces between the aggregate, thereby increasing the compressive strength of the soil or sand mixture (Stocks-Fischer 1999). Most soil is rich with ureolytic bacteria which can precipitate calcite when the right mixture of nutrients and minerals are added (Gomez 2014). Here I explore data sets of soil morphology, chemistry and climate to understand the applicability of this hyperlocal technology at a global scale and share them through an open source geographic information system (GIS).

With knowledge of local soil properties, both mineral and microbial, communities across the earth can implement their own recipes for bioaugmentation to repair, enhance or build earth or concrete structures in a sustainable and low cost manner.
Nearly 3.5 billion people live and work in earthen structures each day. While these structures are extremely functional and long lasting, climate change is altering the environment in which earthen construction techniques work best. New techniques are needed to make this ancient material more resistant to rain and wind. MICP has been used to stabilize historical structures and could be used to reinforce inhabited earthen buildings to make them more resilient in the face of a changing climate.
Soil pH is possibly the most critical soil property for both locating local soil bacteria capable of calcite precipitation and building with an earthen material compatible with MICP. (image Meng 2011)
Earthen construction is also highly susceptible to seismic activity. This is one reason many communities in at risk zones are working to replace their adobe or rammed earthed buildings with concrete. However concrete is typically far more expensive than earth and comes with the added cost of high embodied carbon. Biocementation processes may improve seismic safety in areas where the soil used for construction has a low bearing capacity.
Open source data sets exist for most of the critical soil parameters which play a roll in MICP viability and compressive strength.
Soil pH - while soil pH can be adjusted, a naturally alkaline environment is more likely to harbor ureolytic bacteria.
Soil bulk density - from bulk density and clay/sand content, compressive properties can be predicted.
Clay content
Sand content
Soil depth
Calcium and gypsum toxicity in soil - Locally occurring calcium reduces the need for processed inputs.
Ecological zones - temperature and humidity

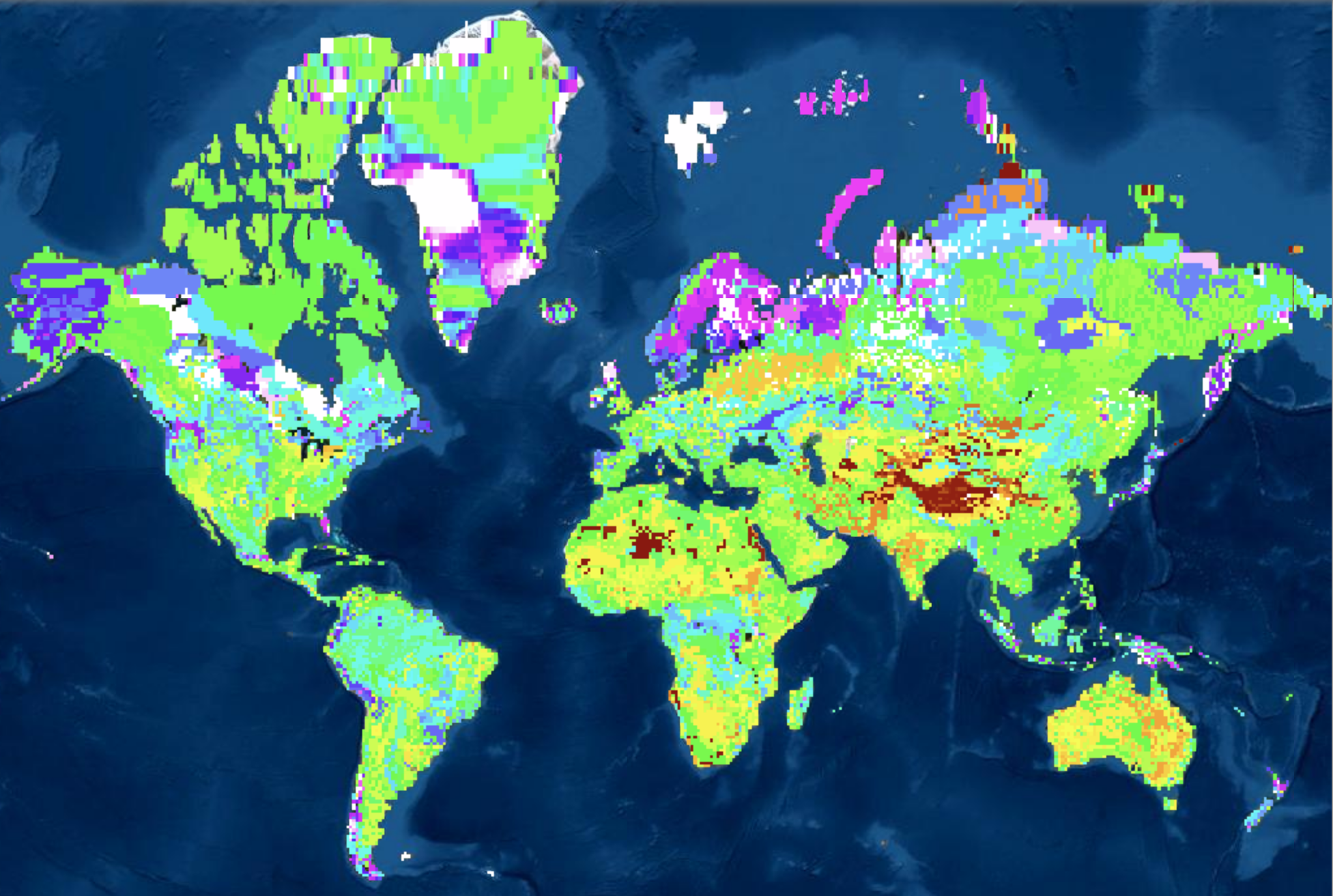
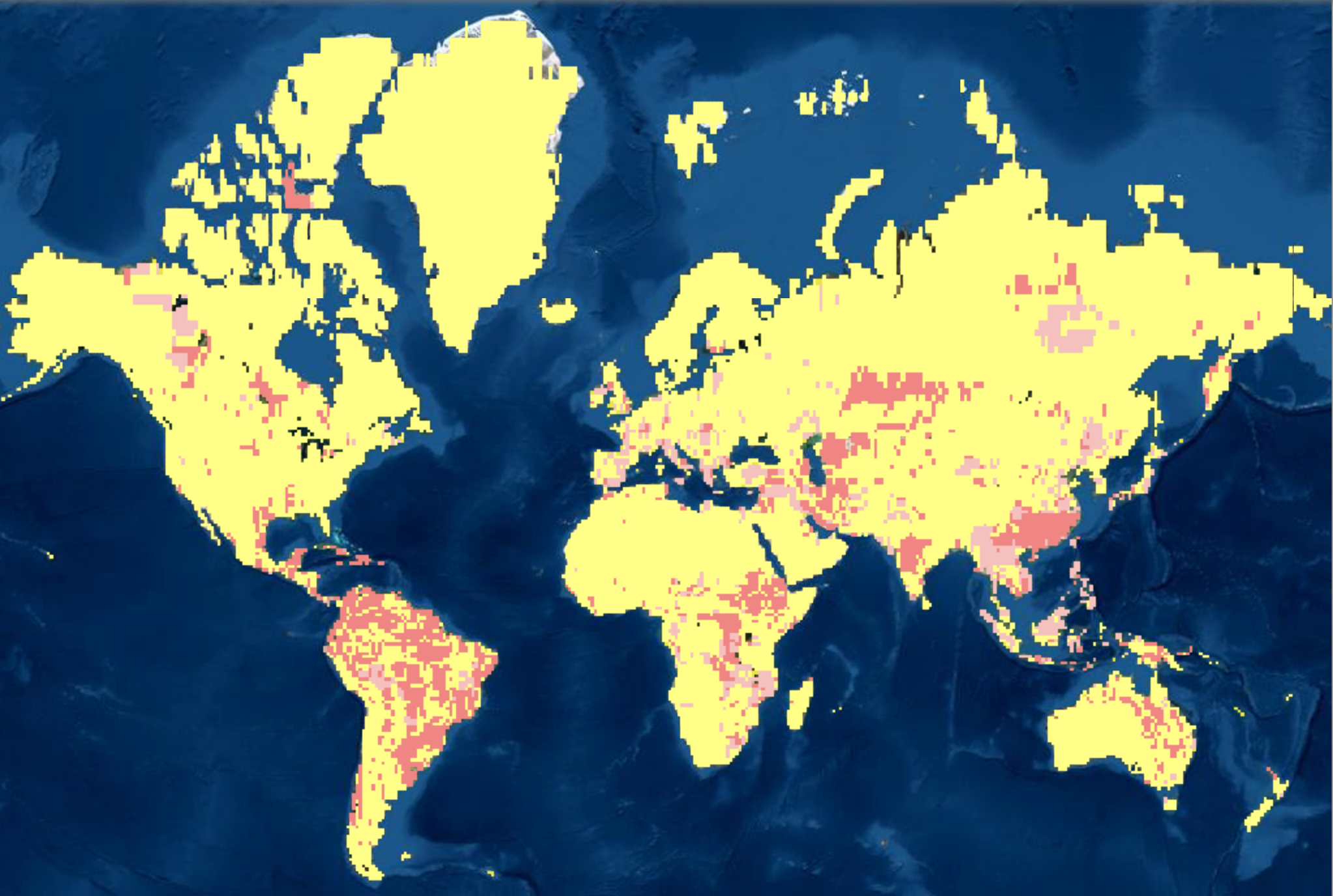

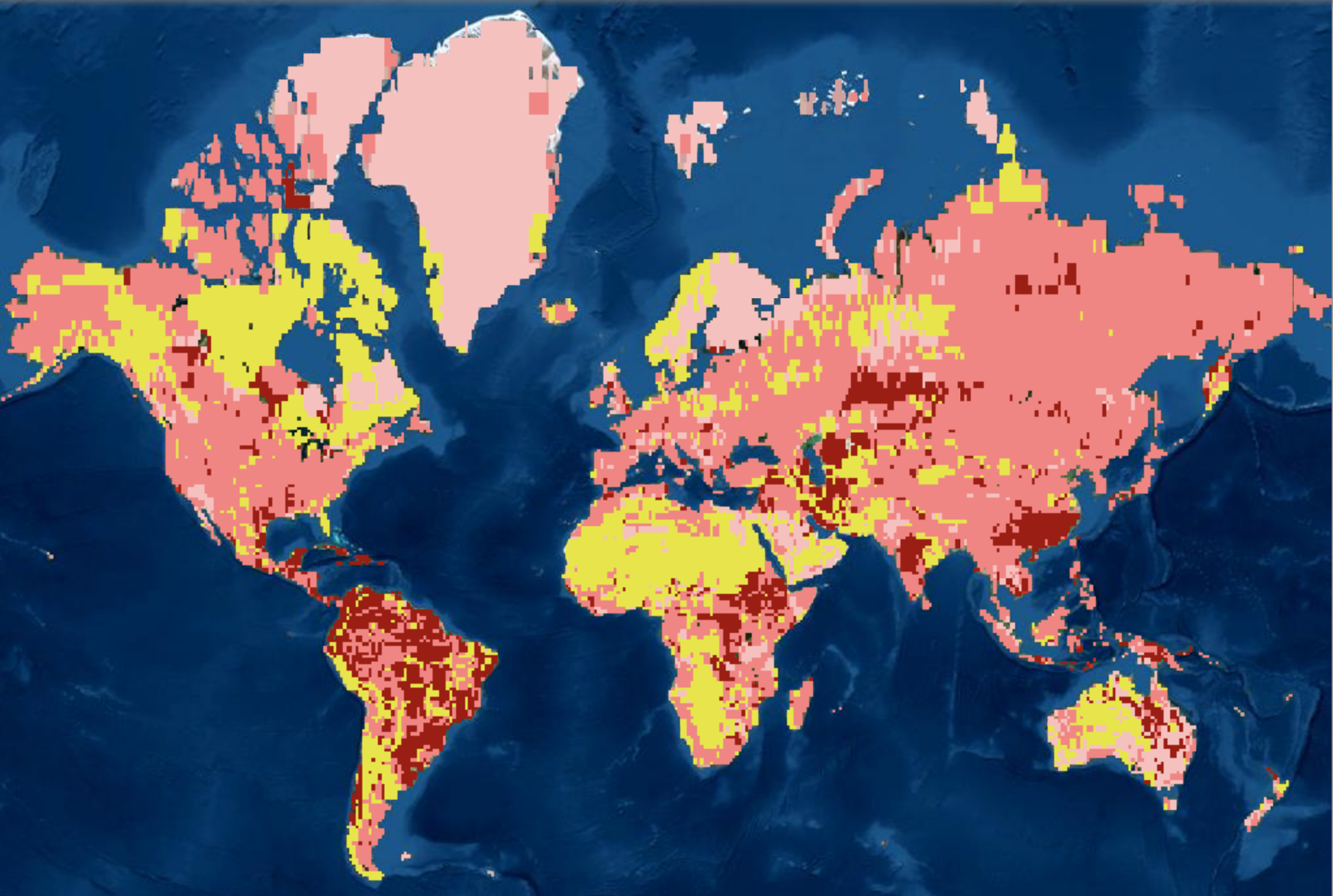
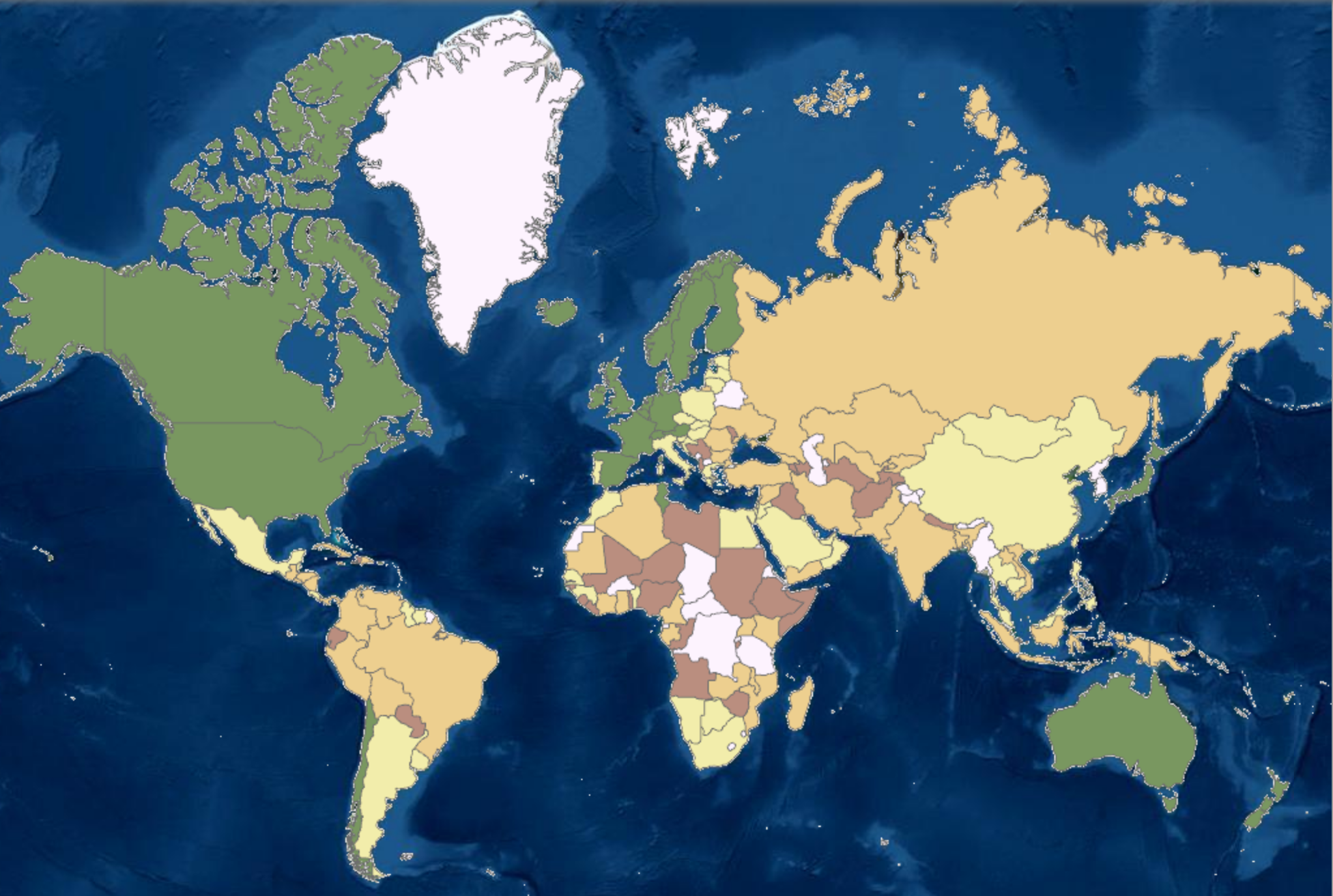
Key considerations can be compared at a local level. For instance alkaline soils (dark red) may be located in regions with a high calcium content, potentially ideal conditions for the sourcing of super local, low embodied carbon building materials.
The database can be explored HERE. I will continue to update it.
References
Gomez, Michael G., Collin M. Anderson, Charles M. R. Graddy, Jason T. DeJong, Douglas C. Nelson, and Timothy R. Ginn. 2017. “Large-Scale Comparison of Bioaugmentation and Biostimulation Approaches for Biocementation of Sands.” Journal of Geotechnical and Geoenvironmental Engineering 143 (5): 04016124. https://doi.org/10.1061/(ASCE)GT.1943-5606.0001640.
“Harmonized World Soil Database v1.2 | FAO SOILS PORTAL | Food and Agriculture Organization of the United Nations.” n.d. Accessed November 2, 2021. https://www.fao.org/soils-portal/data-hub/soil-maps-and-databases/harmonized-world-soil-database-v12/en/.
Macabuag, Joshua, and Subhamoy Bhattacharya. 2008. “Extending the Collapse Time of Non-Engineered Masonry Buildings Under Seismic Loading” 86 (April).
Meng, Lingbo, P. Hess, Natalie Mahowald, Joseph Yavitt, William Riley, Z. Subin, David Lawrence, S. Swenson, Jyrki Jauhiainen, and Daniel Fuka. 2011. “Sensitivity of Wetland Methane Emissions to Model Assumptions: Application and Model Testing against Site Observations.” Biogeosciences Discussions 8 (June). https://doi.org/10.5194/bgd-8-6095-2011.
“Mineral Resources for Earth Architecture | Maps | Data Basin.” n.d. Accessed November 2, 2021. https://databasin.org/maps/8cc34037adb84337bf7fda3d4a7593f7/active/.
Nayfach, Stephen, Simon Roux, Rekha Seshadri, Daniel Udwary, Neha Varghese, Frederik Schulz, Dongying Wu, et al. 2021. “A Genomic Catalog of Earth’s Microbiomes.” Nature Biotechnology 39 (4): 499–509. https://doi.org/10.1038/s41587-020-0718-6.
Shannon Stocks-Fischer, Johnna K. Galinat, and Sookie S. Bang. 1999. “Microbiological Precipitation of CaCO3.” Soil Biology and Biochemistry 31: 1563–71.
Silva, Rui A., Paul Jaquin, Daniel V. Oliveira, Tiago F. Miranda, Luc Schueremans, and Nuno Cristelo. 2014. “Conservation and New Construction Solutions in Rammed Earth.” In Structural Rehabilitation of Old Buildings, edited by Aníbal Costa, João Miranda Guedes, and Humberto Varum, 77–108. Building Pathology and Rehabilitation. Berlin, Heidelberg: Springer. https://doi.org/10.1007/978-3-642-39686-1_3.
“Soil Geographic Databases.” n.d. Www.Isric.Org. Accessed November 2, 2021. https://www.isric.org/explore/soil-geographic-databases.
“WGI 2021 Interactive > Interactive Data Access.” n.d. Accessed November 2, 2021. https://info.worldbank.org/governance/wgi/Home/Reports.